Configuration of the HADES experiment
HADES comprises six identical detector systems arranged as the segments of a circle. This setup enables the detection of the majority of the particles produced during a collision in the target.
In total the HADES experiment consists of more than 82,000 individual detectors. During measurement operation HADES yields as much as 400 MB of data per second, the equivalent of a text document of 200,000 pages. In order to learn more about the structure of protons and neutrons, researchers hunt through this huge volume of data for signs of electron-positron pairs.
The target and start detector
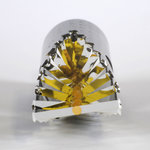
The target is positioned immediately upstream of the start detector. Ions are accelerated to around 90 percent of the speed of light in the UNILAC linear accelerator and the SIS ring accelerator. The target consists of 15 sections of stretched metal foil positioned one after another. It is here that the accelerated ions collide with other atoms, thereby giving rise, ideally, to a brief overlap of the nucleon shells.
In order to be able to measure the speed of the particles created, the start detector assigns a signal to each beam particle as it flies through the target. A diamond detector behind the target serves to separate the signals once more. After all, not every beam particle manages to interact with the atoms in the target and produce the desired decay products.
The RICH detector
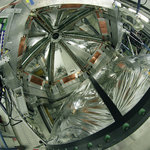
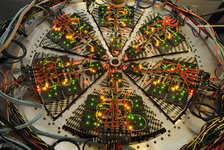
The Ring Imaging Cherenkov detector (RICH) is the centrepiece of the HADES experiment. The RICH detector serves to detect the electrons and positrons that arise when two atomic nuclei collide. It filters these particles out from among the many other reaction products. The detector comprises a chamber filled with perfluorbutane (C4F10) gas. Electrons and positrons travel through this gas faster than light, causing them to emit a special form of light known as Cherenkov radiation. This enables scientists to distinguish electrons and positrons from other, slower particles.
Drift chambers

The initial part of the magnetic spectrometer comprises four so-called multiwire drift chambers (MDCs). These chambers are filled with gas (helium and butane) and traversed by a multitude of fine anode wires, around which an electric field forms. As charged particles — be these electrons or heavy fragments of the atomic nucleus — fly through a chamber, they ionize some of the gas atoms, thereby triggering a signal at an adjacent anode wire.
Each chamber holds six trapezoidal modules mounted on top of one another, each of which in turn consists of several layers of stretched wires. Arranging the wires in a crisscross pattern means that a number of signals overlap, thereby showing where a charged particle has traversed the MDC.
Based on the drift time (the time from the ionization of a gas atom to the triggering of an electric signal at the anode wire), the path of a particle can be tracked with an accuracy of a fraction of a millimetre. This extreme precision is required in order to determine the angle at which the particle leaves the target.
HADES is equipped with four MDCs — two upstream and two downstream of a superconducting magnet.
The superconducting magnet
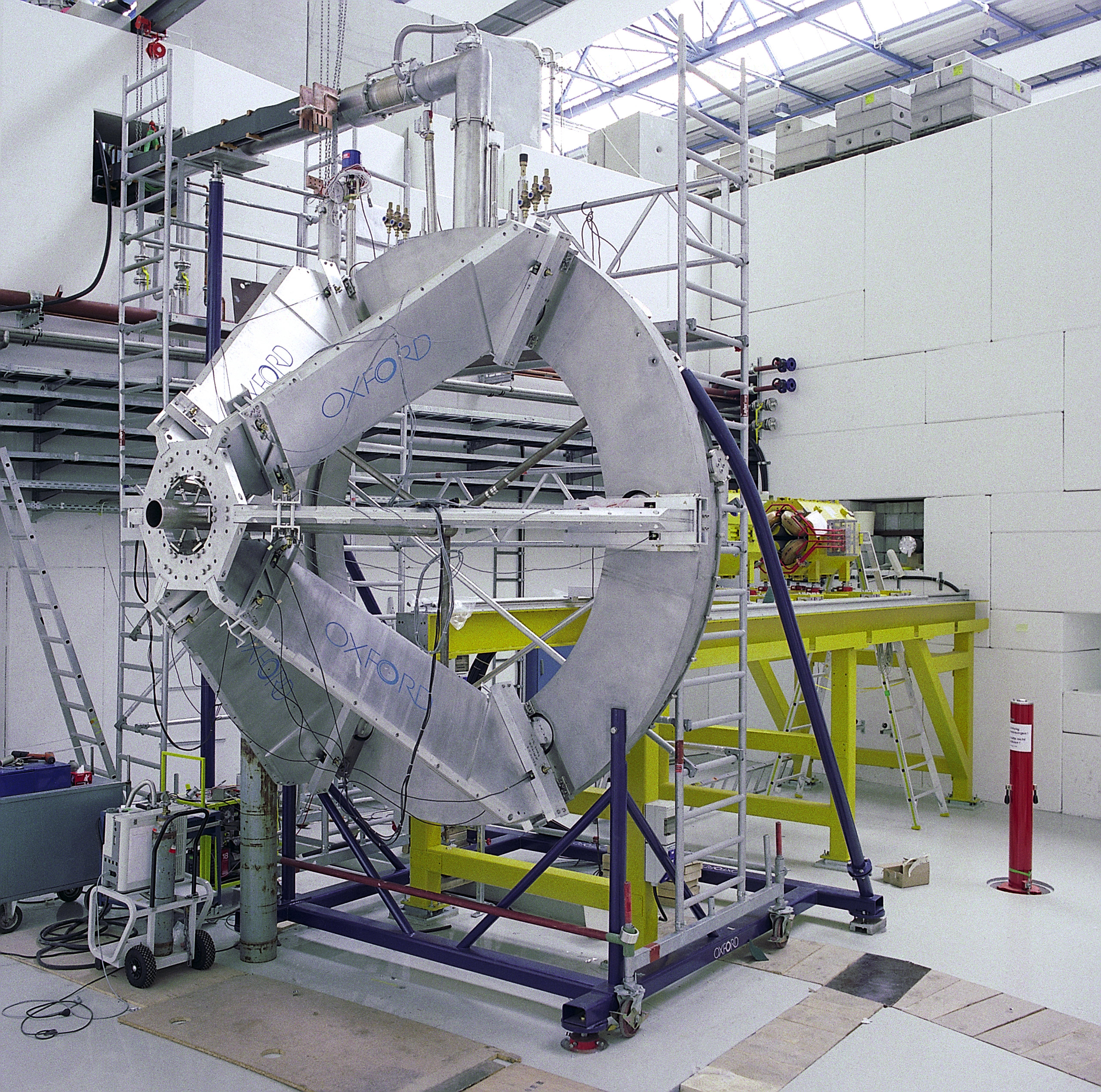
By means of the MDCs and a superconducting magnet consisting of six coils arranged in a ring (torous), it is possible to determine the momentum of all the charged particles produced during the collision. This is important for later identification of the particles.
The magnet generates a ring-shaped field, which exerts a force on any charged particle passing through, thus deflecting the particle onto a different flight path. The angle of this deviation corresponds to the change in momentum. This angle can be determined using the MDCs, which detect the position of the electron before and after the magnet.
To generate as powerful a magnetic field as possible, the magnet is cooled by means of liquid helium to a temperature of 4.7 kelvin (-268.3 °Celsius). This reduces the electrical resistance of the magnet coils to zero, thereby ensuring that all of the electrical power is used to generate the magnetic field.
The time-of-flight wall
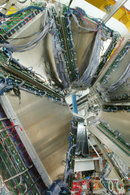
This piece of equipment serves to measure the time it takes for a particle to travel from the start detector at the target to the TOF (time-of-flight) wall, thus enabling the particle’s speed to be calculated. Basically, it is like using a stopwatch. As the particle passes the start detector, a signal is produced. The time until it hits the TOF wall is then measured.
Typically, electrons take around seven nanoseconds from the target to the TOF wall, protons 15 to 20 nanoseconds. Together with the momentum, measured by means of the MDCs and the superconducting magnet, this information permits differentiation and identification of the particles.
The pre-shower detectors

In order to be able to distinguish electron-positron pairs from other particles without errors, a further piece of equipment is required. Pre-shower detectors serve to unambiguously detect and identify electrons and positrons once again, despite the fact that their occurrence, among all the other particles that arise in the reaction, amounts to a mere one-hundredth of a percent.
The pre-shower detectors essentially consist of a number of detector systems interleaved with layers of lead. When an electron or a positron strikes a layer of lead, the particle decays into a gamma particle and an electron, which in turn decays when it strikes the next layer of lead. This shower is only produced by electrons and positrons. Other particles, such as protons, pass through the lead without producing such showers.