Treatment Planning and Validation
Group leader: PD Dr. Michael Krämer
Main research topics
- Macroscopic dose distribution
- Adaptive treatment planning
- "Kill-painting" (e.g. to treat hypoxic tumours)
- Mathematical optimization algorithms
- Treatment planning with TRiP98 for combined multi-ion treatments
- "Space TRiP": adaptation of radiotherapy planning concepts for space radiation protection applications, extension of TRiP98 to higher (FAIR) energies
- Microscopic Monte Carlo calculations
- Low energy electron interactions
- Radiation chemistry under ultra-high dose rate irradiation
- Oxygen Effect
- Nanoparticle radiation enhancement and electron emission from solids
- Experimental validation of treatment planning
- Verification of 3D dose distributions
- Biological verification using gel-based 3D bio-phantoms
- Biological phantoms with different oxygen concentrations
- Tests of adaptive treatment plans
Macroscopic dose distribution
On the macroscopic scale, mainly for the purpose of treatment planning, we run a homegrown numerical transport model. It considers electromagnetic and nuclear interactions with empirical corrections for the attenuation of the primary beam as well as for the creation of nuclear fragments. This model is implemented in our treatment planning code, TRiP98. We further use the Local Effect Model (LEM) to calculate and optimize RBE-weighted (“biological”) dose distributions.
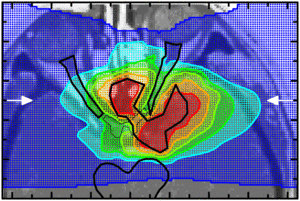
TRiP98 was in clinical use in the GSI pilot project, and Siemens chose it as a prototype for their commercial SynGo PT TPS. Nowadays the continuously developing code serves as a research prototype inside and outside GSI to support new developments in ion beam radiotherapy. Recently support for alternative ions such as 16O [4] and 4He [3] has been added, and the use of different ions can be optimized in a single plan [7]. Moreover, optimization algorithms have been enhanced with the oxygen enhancement ratio (OER) as a driving force to allow the treatment of hypoxic tumour microenvironments via "kill-painting" [2]
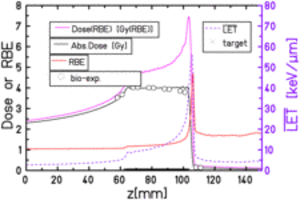
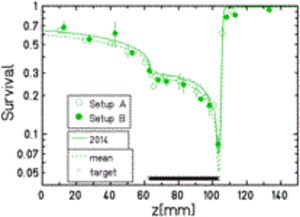
The most recent extension [5], is aimed at space radiation research, in particular shielding of space vessels and habitats, thus called SpaceTRiP. Just as in radiotherapy, the target geometry is described by regular cubic voxels, but with different material composition. The internal transport code of TRiP98 is being extended to meet additional requirements, i.e. projectiles up to iron, energies up to 1 GeV/n and above, various target materials etc.
Microscopic Monte Carlo calculations
On the microscopic/nanoscopic scale one has to consider elementary interactions to describe energy and damage deposition. For this purpose, we use the homegrown Monte Carlo code TRAX which describes the production and transport of secondary electrons produced by heavy ions in matter.

Radiation chemistry has been implemented in its recent extension TRAX-CHEM [6] which enables the simulation of the prechemical and chemical stage of radiation interaction in pure or oxygenated [8] water targets. This new functionality is being applied to investigate radiosensitization by nanoparticles, the variation of the Oxygen Enhancement Ratio seen with high LET radiation, and tissue sparing at ultra-high dose rate irradiation (FLASH effect) amongst others.
![Fig.4: Nanoscale dose and dose enhancement factor distribution in perpendicular (r) and longitudinal (z) direction of a water-embedded gold nanoparticle (7 nm radius) for 44 MeV/u C ions [9]. Photo: M. Krämer, GSI Helmholtzzentrum für Schwerionenforschung GmbH](/fileadmin/_processed_/6/3/csm_Dose_Differnce_Bild_5_edb95ef9b5.jpg)
![Fig.5: G-values for the production of superoxide and perhydroxyl obtained with TRAX-CHEM (left) and OER (right) as a function of target oxygenation [8]. Photo: M. Krämer, GSI Helmholtzzentrum für Schwerionenforschung GmbH](/fileadmin/_processed_/e/9/csm_Protons_Bild6_c127e76e88.jpg)
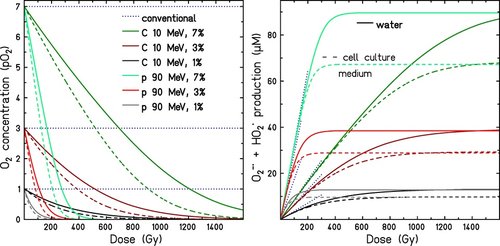
Experimental validation of treatment planning
Experimental validation of treatment planning
We do not rely solely on simulations, but regularly perform radiobiological experiments in order to verify our predictions. The classical tools are cellular phantoms such as stacks and the Biophantom in order to measure one- and twodimensional distributions of cell survival under patient-like conditions. Currently, we are exploring gel-based 3D-phantoms for treatment plan verification experiments in normoxic conditions.
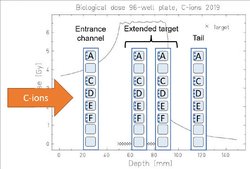
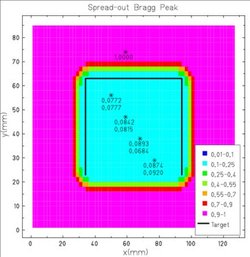
The so-called hypoxia chambers allow irradiations under controlled oxygen concentrations. They have been used to verify the "kill-painting" approach as a proof of concept [1,4].

